FHV-1 enters the cell by receptor-mediated endocytosis
Authors: Aleksandra Synowiec https://orcid.org/0000-0001-9846-6922 a.synowiec@doctoral.uj.edu.pl, Agnieszka D?browska https://orcid.org/0000-0003-1499-4950, Magdalena Pachota https://orcid.org/0000-0001-5520-6979, Meriem Baouche https://orcid.org/0000-0002-7344-1769, Katarzyna Owczarek https://orcid.org/0000-0002-2166-4688, Wojciech Ni?ański https://orcid.org/0000-0003-1056-0784, Krzysztof Pyrc k.a.pyrc@uj.edu.plAUTHORS INFO & AFFILIATIONS
DOI: https://doi.org/10.1128/jvi.00681-23
ABSTRACT
Feline herpesvirus type 1 (FHV-1) is an enveloped dsDNA virus belonging to the Herpesviridae family and is considered one of the two primary viral etiological factors of feline upper respiratory tract disease. In this study, we investigated the entry of FHV-1 into host cells using two models: the AK-D cell line and primary feline skin fibroblasts (FSFs). We employed confocal microscopy, siRNA silencing, and selective inhibitors of various entry pathways. Our observations revealed that the virus enters cells via pH and dynamin-dependent endocytosis, as the infection was significantly inhibited by NH4Cl, bafilomycin A1, dynasore, and mitmab. Additionally, genistein, nystatin, and filipin treatments, siRNA knock-down of caveolin-1, as well as FHV-1 and caveolin-1 colocalization suggest the involvement of caveolin-mediated endocytosis during the entry process. siRNA knock-down of clathrin heavy chain and analysis of virus particle colocalization with clathrin indicated that clathrin-mediated endocytosis also takes part in the primary cells. This is the first study to systematically examine FHV-1 entry into host cells, and for the first time, we describe FHV-1 replication in AK-D and FSFs.
IMPORTANCE
Feline herpesvirus 1 (FHV-1) is one of the most prevalent viruses in cats, causing feline viral rhinotracheitis, which is responsible for over half of viral upper respiratory diseases in cats and can lead to ocular lesions resulting in loss of sight. Although the available vaccine reduces the severity of the disease, it does not prevent infection or limit virus shedding. Despite the clinical relevance, the entry mechanisms of FHV-1 have not been thoroughly studied. Considering the limitations of commonly used models based on immortalized cells, we sought to verify our findings using primary feline skin fibroblasts, the natural target for infection in cats.
INTRODUCTION
Herpesviruses constitute a large family of enveloped, double-stranded DNA viruses that infect a wide range of animals. Following the initial infection, herpesviruses possess the ability to remain in the cell in a dormant state, causing lifelong, latent infection (1, 2). Among the most prevalent and best-studied herpesviruses are the human herpes simplex viruses (HSV), causing cold sores and genital lesions (3). However, animal viruses are also getting attention, as they cause economic losses or affect companion animals. To make an example, pseudorabies is the etiological agent of Aujeszky’s disease, causing respiratory disorders, abortions, and neurological disease in pigs (4); bovine herpesvirus-1 causes conjunctivitis, pneumonia, genital disorders, abortions, and upper respiratory infections in cattle (5); equine herpesvirus-1 (EHV-1) causes respiratory disease, abortions, and, in some cases, neurological disease in horses (6); and canid herpesvirus-1 is responsible for neonatal mortality, ocular, and respiratory disorders in canines (7, 8).
Feline herpesvirus type 1 (FHV-1) belongs to the subfamily Alphaherpesvirinae under the genus Varicellovirus (9). The virus primarily infects domestic cats, but lions and cheetahs are also susceptible (10, 11). FHV-1 is responsible for feline viral rhinotracheitis, which accounts for over half of viral upper respiratory diseases (URTD) in cats and ocular lesions that may result in loss of sight (12). While the disease course is primarily mild, FHV-1 infections may also cause severe symptoms, including pneumonia, gastritis, and necrotizing bronchiolitis accompanied by diffuse fibrinonecrotic bronchopneumonia (13 - 17). Further, bacterial and viral superinfections are common in cats with URTD and may have fatal outcomes if not managed. Similarly to other alphaherpesviruses, cats become persistently infected following the initial exposure to the virus, and the infection may reactivate under stress or immune deficits (1, 2).
During the infection, herpesviruses usually utilize heparan sulfate proteoglycans (HSPGs) to attach to the susceptible cell (18, 19) via glycoprotein B (gB), which acts as a fusogen, and glycoprotein C (gC) (20), which enables or enhances the virus interaction with the entry receptor. Glycoprotein D (gD) is believed to mediate the entry of herpesviruses, interacting with the entry receptor. The binding of the gD results in conformational changes of the entry complex involving also gH/gL viral glycoproteins. This triggers a series of events, which eventually result in the activation of the gB protein, which facilitates viral entry (21). There is a plethora of cell factors that were described to serve as such, e.g., HSV-1 utilizes the herpesvirus entry mediator (HVEM, HveA) (22), a member of the immunoglobulin family; nectin-1 (HveC) (23); and 3-O-sulfonated heparan sulfate (18, 24). The viral DNA is delivered to the cell following receptor binding, internalization, and fusion. Interestingly, herpesviruses use different internalization routes depending on the cell and tissue type ( 7, 21, 25 - 27). FHV-1, similarly to other herpesviruses, attaches to the cell surface using HSPGs (28, 29), but the entry mechanisms have not been studied in detail.
In this work, we made an effort to elucidate the mechanisms of FHV-1 entry into susceptible cells. To ensure the relevance of the results, except for the studies on well-established cell line AK-D, we also employed the primary feline skin fibroblasts (FSFs), the natural target for the infection in vivo. Using these models, we mapped the early stages of viral infection. We confirmed that FHV-1 uses HSPGs as adhesion factors, and this process is mediated by viral glycoprotein C. Upon the viral adhesion to the host’s cell surface, the virus interacts with the receptor(s), which remains unidentified. FHV-1 is further internalized via pH-dependent and caveolin-mediated endocytosis. Our findings shed light on the biology of the FHV-1 entry and may support future efforts to limit the virus transmission or develop novel antivirals.
MATERIALS AND METHODS
Mammalian cell culture
Crandell-Rees feline kidney (CRFK, ATCC: CCL-94) cells were maintained in Dulbecco-modified Eagle’s medium (DMEM, high glucose, Life Technologies, Poland) supplemented with 5% heat-inactivated fetal bovine serum (FBS, Life Technologies, Poland) (5% DMEM). Feline fetal lung cells (AK-D, ATCC: CCL-150) were maintained in Ham’s F-12K (Kaighn’s) Medium (Life Technologies, Poland) supplemented with 10% heat-inactivated fetal bovine serum. Media were supplemented with penicillin (100 U/mL; Thermo Fisher Scientific) and streptomycin (100 μg/ml; Thermo Fisher Scientific), termed 1% P/S. Cells were cultured at 37°C, under 5% CO2. All cells were regularly tested for mycoplasma.
Insect cell culture
Sf9 (Spodoptera frugiperda, ATCC: CRL-1711) and HF (High Five, Trichoplusia ni, ATCC: CRL-7701) cells were cultured in Insect Cell Culture Medium (ESF 921, Expression Systems, CA, USA) medium supplemented with 2% FBS + 1% P/S, additionally supplemented with 10 μg/mL gentamycin (Gibco, Thermo Fisher Scientific, Poland) and 0.25 μg/mL amphotericin B (Gibco, Thermo Fisher Scientific, Poland). The cells were maintained in a humidified incubator at 27°C. Sf9 cells were used for baculovirus generation and amplification, whereas HF cells were used for protein expression.
Feline skin fibroblast isolation and culture
To isolate cat fibroblast, wedge biopsy of skin from the groin region was taken from two cats (ages 2.5 and 3 years) during a routine operation; the cats were patients of the Department of Reproduction and Clinic of Farm Animals in Wroclaw. Samples were collected under the decision of the Local Ethical Committee for Animal Research in Wroclaw, Poland, dated 14 October 2014.
Tissues were placed in falcon tubes containing phosphate-buffered saline (PBS) (Sigma-Aldrich, Poland) supplemented with 1% P/S. The tissue samples were rinsed twice with cold PBS and then put in a sterile culture dish (Nunclon Delta Surface, Thermo Fisher Scientific, Denmark). The tissue was minced into 1 mm2 using #10 bistoury blade and cultured in 12-well plates in low-glucose DMEM (LG-DMEM; Merck KGaA, Darmstadt, Germany) supplemented with 10% FBS and 1% P/S. Half of the medium was refreshed after 24 h, and the complete medium was refreshed every 3 days. The primary cultures were established by the outgrowth of primary fibroblast from explanted tissue pieces. After the isolation, feline skin fibroblasts were cultured in DMEM supplemented with 10% FBS, 1% P/S, 1% GlutaMAX (Thermo Fisher Scientific, Poland), 1% Non-Essential Amino Acids (NEAA, Thermo Fisher Scientific, Poland), 1% HEPES (Thermo Fisher Scientific, Poland), and Fibroblast Growth Factor-Basic (bFGF, 2.5 ng/mL; Sigma-Aldrich, Poland).
Virus
Virus stocks were generated by inoculating fully confluent CRFK cells with feline herpesvirus type 1 strain C-27 (FHV-1 C-27; ATCC: VR-636) at 1,000 50% tissue culture infectious dose/mL (TCID50/mL). Forty-eight hours post-inoculation (hpi), virus-containing supernatants were collected, aliquoted, and stored at ?80°C. Infectious samples were titrated as previously described (30). All viral work was performed under biosafety level 2 conditions.
Inhibitors
All the inhibitors were purchased from Sigma-Aldrich (Poland), except for ammonium chloride which was purchased from Bioshop, Poland. The chemical inhibitors used in this study are listed in Table 1.
TABLE 1
TABLE 1 Chemical inhibitors used in the study
Chemical inhibitor | Mechanism of action | Concentration used in this study |
|
AK-D cells | FSF cells |
|
|
|
NH4Cl | Endosome alkalizing agent (31) | 200 mM | 100 mM |
|
Bafilomycin A1 | ATPase inhibitor (32) | 200 nM | 200 nM |
|
Genistein | Blocks caveolin-dependent endocytosis (33) | 800 μM | 800 μM |
|
Nystatin | Disrupts caveolae, sterol-binding agent (34) | 75 μM | 75 μM |
|
Filipin III | Cholesterol-binding agent (34) | 2 μg/mL | 2 μg/mL |
|
Dynasore | Inhibits GTPase activity and thus dynamin activity (35) | 400 μM | 400 μM |
|
Mitmab | Inhibits dynamin activity (36) | 40 μM | 40 μM |
|
Camostat | Serine proteases inhibitor (37) | 400 μM | 400 μM |
|
E64d | Cysteine proteases inhibitor (38) | 20 μM | 20 μM |
|
Heparan sulfate | Mimicks adhesion molecule (18) | 1,000 μg/mL | 1,000 μg/mL |
|
Genetic constructs (plasmids and bacmids)
The codon-optimized (insect cells) genes coding for glycoprotein B, glycoprotein C, and glycoprotein D, each tagged with 6× His-tag together with hemagglutinin (HA)-tag (YPYDVPDYA) proteins, were synthesized (GeneArt, Thermo Fisher Scientific, Erlangen, Germany), delivered in pMA plasmids, and subcloned to pFastBac Dual plasmids (Thermo Fisher Scientific, Poland), under the control of the polyhedrin promoter. Recombinant bacmids and baculoviruses were generated using the BAC-TO-BAC system (Thermo Fisher Scientific, Poland), according to the manufacturer’s protocol. Briefly, the pFastBac Dual recombinant plasmids were transformed into MAX Efficiency DH10Bac cells (Thermo Fisher Scientific, Poland), and positive colonies were verified by colony PCR and sequencing; bacmid DNA from selected clones was isolated. The bacmids were then transfected into Sf9 cells. Five days post-inoculation (p.i.), p1 recombinant baculovirus stocks were harvested and amplified in infected Sf9 cells, final p2 recombinant baculovirus stocks were collected, and viral titers were assessed using the plaque assay.
Protein expression and purification
The HF cells were harvested by cetrifugation (500 × g, 5 min) and resuspended, then infected with p2 baculovirus stocks at a multiplicity of infection (MOI) of 1 or 5 and cultured until 96 hpi. The supernatants and cells were harvested every 24 h, and the expression and secretion of proteins were verified by gel electrophoresis and western blot. Subsequently, proteins were purified by Ni2+ affinity chromatography (Bio-Rad, Warsaw, Poland). First, proteins were mixed 1:1 (vl/vol) with a binding buffer (500 mM NaCl, 50 mM Tris, 5 mM imidazole, 5% glycerol, pH 7.5) and loaded on a column. The column was washed five times with 20 mL of washing buffer (500 mM NaCl, 50 mM Tris, 25 mM imidazole, 5% glycerol, pH 7.5), and the purified protein was eluted in 5 mL of elution buffer (500 mM NaCl, 50 mM Tris, 500 mM imidazole, 5% glycerol, pH 7.5) divided into five elution fractions (1 mL each). Fractions were analyzed by western blot.
Western blot analysis
Cells were harvested in Radioimmunoprecipitation Assay (RIPA) buffer (50 mM Tris, 150 mM NaCl, 1% Nonidet P-40, 0.5% sodium deoxycholate, 0.1% SDS, pH 7.5) supplemented with 0.5 M EDTA and protease inhibitors cocktail (cOmplete Tablets, Roche, Poland) and incubated for 20 min at 4°C. Protein concentration was assessed with Pierce BCA Protein Assay Kit (Thermo Fisher Scientific, Poland), according to the manufacturer’s protocol. Samples containing equal amounts of proteins were mixed with SDS-PAGE sample buffer (0.5 M Tris, pH 6.8, 10% SDS, 50 mg/mL Dithiothreitol (DTT)), denatured for 10 min at 95°C, and separated by SDS-PAGE alongside dual-color PageRuler prestained protein size markers (Thermo Fisher Scientific, Poland). Subsequently, proteins were electrotransferred onto a polyvinylidene difluoride membrane (GE Healthcare, Poland) for 70 min at 100 V in transfer buffer (25 mM Tris, 192 mM glycine, 20% methanol) at 4°C. The non-specific binding sites on the membrane were blocked overnight at 4°C with 10% milk (Bioshop, Poland) in Tris-buffered saline supplemented with 0.25% TWEEN-20 (Bioshop, Poland) (TBST). Subsequently, membranes were incubated with primary antibodies specific to HA-tag produced in rabbits (Sigma-Aldrich, Poland) diluted 1:1,000 in 2.5% milk in TBST and incubated for 2 h at room temperature. After being washed in TBST thrice, the membrane was incubated with HRP-labeled anti-rabbit IgG antibody (RRID: AB_257896, Sigma Aldrich, Poland) diluted 1:20,000 in 2.5% milk in TBST for 1 h at room temperature. Finally, the proteins were visualized using the ECL system (Amersham, Poland). All antibodies used for western blotting are listed in Table 2.
TABLE 2
TABLE 2 Antibodies used in this study
Antibody | Provider | Catalog no. | Immunostaining dilution | Western blot dilution |
Rabbit anti-caveolin-1 | Cell Signaling | D46G3 | 1:200 | 1:1,000 |
Rabbit anti-clathrin | Cell Signaling | P1663 | 1:200 | 1:1,000 |
Rabbit anti-EEA1 | Cell Signaling | C45B10 | 1:100 | Not used |
Rabbit anti-α-tubulin | Santa Cruz | sc-5546 | Not used | 1:200 |
Mouse anti-FHV-1 | Bio-Rad | MCA2490 | 1:500 | Not used |
Donkey anti-rabbit Alexa Fluor 488 | Thermo Fisher Scientific | A-21206 | 1:400 | Not used |
Donkey anti-mouse Alexa Fluor 488 | Thermo Fisher Scientific | A-21202 | 1:400 | Not used |
Donkey anti-mouse Alexa Fluor 546 | Thermo Fisher Scientific | A-10036 | 1:400 | Not used |
HRP-labeled anti-rabbit antibody | Sigma-Aldrich | AB_257896 | Not used | 1:20,000 |
HRP-labeled anti-mouse antibody | Dako | P0214 | Not used | 1:20,000 |
Rabbit anti-HA-tag | Sigma-Aldrich | H6908 | 1:500 | 1:1,000 |
Immunostaining and confocal microscopy
Cells were seeded on the coverslips in a 12-well cell culture plate and cultured in a standard medium for 24 h at 37°C. Upon experimental procedure, the cells were fixed with 4% paraformaldehyde in PBS for 15 min at room temperature. Subsequently, cells were washed with PBS and permeabilized with 0.5% Triton X-100 (Bioshop, Canada) for 12 min at room temperature. Afterward, non-specific binding sites were blocked by overnight incubation at 4°C in 10% Bovine Serum Albumin (BSA, Bioshop, Canada). The next day, cells were incubated for 2 h with mouse primary monoclonal anti-FHV antibodies to visualize viral particles. To visualize feline proteins, slides were incubated with primary antibodies against caveolin-1, clathrin, or early-endosome antigen 1 (EEA1), diluted in 2.5% BSA in PBS, together with anti-FHV antibodies. Antibody specificity was confirmed with isotype control antibodies used at the same concentrations. Subsequently, cells were washed four times with 0.5% Tween (Bioshop, Canada), incubated for 1 h with secondary antibodies coupled with Alexa Fluor, and diluted in 2.5% BSA. When needed, cells were washed four times with 0.5% Tween and incubated for 1 h with phalloidin conjugated with Alexa Fluor 647 (0.2 U/mL, diluted in 2.5% BSA, Invitrogen, Poland) to stain F-actin filaments. Subsequently, cells were washed four times with PBS, and nuclei (DNA) were stained with 4′,6′-diamidino-2-phenylindole (DAPI dye) (0.1 μg/mL, Sigma-Aldrich, Poland) for 20 min at room temperature. Cells were washed twice with PBS, and coverslips with immunostained cells were mounted on glass slides in ProLong Diamond Antifade Mountant medium (Life Technologies, Poland), sealed, and stored at 4°C.
Images were acquired under a Zeiss LSM 710 confocal microscope (Carl Zeiss Microscopy GmbH; release version 8.1) using ZEN 2012 SP1 software (Carl Zeiss Microscopy GmbH; black edition, version 8.1.0.484) and collected through z-stacks (step size 0.20 μm or 0.10 μm, frame size 1,024 × 1,024, pixel size 0.13 μm) unless stated otherwise. Images were processed using the ImageJ FIJI version (National Institutes of Health, Bethesda, MD, USA) and are presented as the maximal projections if not stated otherwise. Colocalization parameters (Pearson’s and Manders’ coefficients) were calculated using the JaCoP plugin (39). All antibodies used for immunostaining are listed in Table 2.
DNA isolation
The Viral DNA/RNA Isolation Kit (A&A Biotechnology) was used to isolate viral DNA from cell culture supernatants, according to the manufacturer’s instructions
Virus entry assay
Fully confluent cells were seeded on 96-well plates 24 h before the inoculation. Before the infection, the supernatant was discarded, and 90 μL of fresh medium supplemented with the inhibitor was added. Plates were incubated for 60 min at 37°C, and subsequently, 10 μL of mock or virus (MOI = 1) was added. Plates were incubated for 1 h (FHV-1) at 37°C, supernatants were discarded, and cells were washed twice with PBS. Finally, a fresh medium without inhibitor was added to each well, and cells were incubated for 8 h. After that time, the supernatants were subjected to DNA isolation and qPCR analysis, cells were fixed and immunostained, and the experimental controls were used for the whole set of inhibitors tested.
Cytotoxicity assay
Cells were cultured on a 96-well plate for 24 h. Afterward, supernatants were discarded, and 100 μL of fresh medium with an inhibitor or mock sample was added to each well. After 48 h at 37°C, cell viability was evaluated using the XTT Viability Assay Kit (Biological Industries, Israel), according to the manufacturer’s protocol. Following 2 h incubation at 37°C, the supernatants were transferred into the transparent 96-well plate, and absorbance (λ = 480 nm) was measured. Obtained results were normalized to the absorbance of the control sample (untreated cells).
siRNA silencing
Cells were seeded into 96-well plates (20,000 cells/well) or 48-well plates (50,000 cells/ well) and incubated for 24 h before the experiment. siRNA transfection was performed 24 h and 48 h later, a seeding to increase knock-down efficiency. Cells were transfected using Invitrogen RNAiMAX Lipofectamine (Thermo Fisher Scientific) system according to the manufacturer’s instructions. Briefly, 1 pmol (96-well plate) or 2.5 pmol (48-well plate) of custom Silencer Select siRNAs was mixed in 10 μL or 25 μL of the OptiMEM (Thermo Fisher Scientific) per well, respectively, then incubated for 5 min at room temperature, and added to cells dropwise. siRNA used in this study was designed to target feline caveolin 1 (NM_001024163.1) and clathrin heavy chain (XM_045044808.1, XM_045044809.1, XM_045044810.1). The siRNA sequences are listed in Table 3. Additionally, Silencer Select Negative Control No. 1 siRNA (Thermo Fisher Scientific) was used as a non-targeting control.
TABLE 3
TABLE 3 The sequences of designed siRNA used in this study
Name | Target mRNA | Sequence 5′ ≥ 3′ |
Felis catus-Cav1-siRNA1 | Feline caveolin 1 | CCUUCACUGUGACAAAAU |
Felis catus-Cav1-siRNA2 | Feline caveolin 1 | GUACAUAACUGAAAAUGU |
Felis catus-Clat-siRNA1 | Feline clathrin | GGAGAAAUGGUUAAAAGAA |
Felis catus-Clat-siRNA2 | Feline clathrin | GGAAAGUAUUGUGAGAAGA |
Quantitative real-time PCR
2.5 μL of isolated DNA or cDNA was amplified in a reaction of 10 μL containing 1 × Kapa Probe Fast qPCR MasterMix (A&A Biotechnology, Poland), 100 nM of specific probe labeled with 6-carboxyfluorescein, and 6-carboxytetramethylrhodamine (5′-TAT ATG TGT CCA CCA CCT TCA GGA TCT ACT GTC GT-3′), together with 450 nM of each sense (5′-AGA GGC TAA CGG ACC ATC GA-3′) and antisense primer (5′-GCC CGT GGT GGC TCT AAA C-3′). Specific probe and primers were used to amplify the 81 bp sequence fragment of the glycoprotein B gene of FHV-1 (40). The reaction was carried out in a thermocycler (CFX96 Touch Real-Time PCR Detection System, Bio-Rad), according to the scheme 3 min at 95°C, followed by 39 cycles of 15 s at 95°C and 30 s at 58°C. To quantify nucleic acids, standards were prepared as described previously (41). Eight 10-fold serial dilutions were used as a template to develop a standard curve.
Statistical analysis
Data are presented as the mean ± SD (standard deviation) from at least three independent experiments (biological repeats). Grubbs’ test (α = 0.05) was used to identify outliers. A P-value <0.05 was considered statistically significant unless stated otherwise in the text.
RESULTS
FHV-1 replicates in feline cell lines and primary feline skin fibroblasts
The kidney-derived cell line (CRFK) is the accepted model to study FHV-1 pathogenesis. However, it is based on the immortalized kidney cells that may affect the entry route of the virus. Hence, parallelly, we evaluated the replication of FHV-1 in fetal feline lung cells (AK-D) and feline primary skin fibroblasts (FSFs) using two different MOIs. We observed abundant viral replication in all tested models (Fig. 1A). The replication kinetics in all tested cell lines were similar, with a single replication cycle lasting for 6–8 h, as previously reported for other herpesviruses (42, 43). The cytopathic effect was evident as early as 6 h post-infection at MOI = 1 (Fig. S1). The efficient attachment to CRFK, AK-D, and FSFs was also confirmed using immunostaining and confocal microscopy analysis (Fig. 1B). AK-D and FSFs cells were used for further analyses due to their relevance.
Fig 1
Fig 1 FHV-1 replicates in feline cells. (A) Infections with FHV-1 at MOI = 0.001 and MOI = 1 were carried out using primary FSF, feline fetal lung cells (AK-D), and CRFK cells. Samples were collected at different time points, subjected to viral DNA isolation followed by qPCR, and are presented as a mean of the number of FHV-1 DNA copies/mL ± SD from at least three independent biological repeats. Data were analyzed with Shapiro-Wilk and Brown-Forsythe tests. The Kruskal-Wallis test with post hoc Dunn’s test was used to determine the significance of differences between compared means. Values statistically significant are indicated by asterisks: *P < 0.05, ***P < 0.001, ****P < 0.0001. (B) FHV-1 replication was also confirmed using confocal microscopy. Blue color denotes nuclei, red F-actin, and green FHV-1 virions. Representative images are shown as maximum projections of XY stacks. Scale bar: 10 μm.
FHV-1 attaches to the cell surface using HSPGs
To assess the FHV-1 entry into the permissive cells, we expressed ectodomains of three viral proteins that may be involved in the process (Fig. 2A). The supernatants from transduced insect cells were collected, and proteins were purified using affinity chromatography. The expression was verified collecting supernatants directly from insect cell, and then analyzed using a western blot with an anti-HA antibody (Fig. 2B). Bands corresponding in size to gB (~110 kDa, 48 kDa), gC (~85 kDa, 65 kDa, 48 kDa, 44 kDa), and gD (63 kDa, 47 kDa) were recorded. Next, we checked whether the expressed proteins bind to the permissive cells by incubating them with purified proteins for 1 h at 37°C. Using confocal microscopy, we observed the adhesion of gC and gD; we were not able to visualize gB (Fig. 2C). gC is believed to interact with HSPGs (29), and we confirmed this by incubating AK-D cells with gC in the presence or absence of soluble HS at 1,000 μg/mL. The glycoprotein was visualized using antibodies against HA-tag and analyzed using confocal microscopy (Fig. 2D). We have also studied the effect of HS on the entry of FHV-1 and observed a vast decrease in viral replication both in FSFs and AK-D cells (Fig. 2E), which may suggest that HS serves as an entry receptor; however, this requires further investigation.
Fig 2
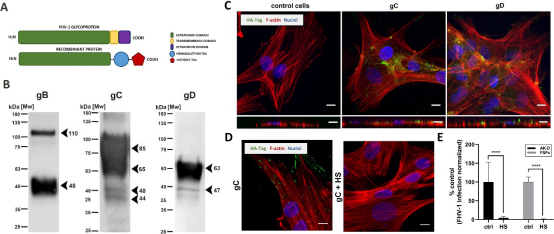
Fig 2 FHV-1 glycoproteins expression and interaction with cell host surface. (A) The scheme of expression constructs. (B) Expression of viral glycoproteins and western blot analysis. Truncated FHV-1 glycoprotein B (gB), glycoprotein C (gC), and glycoprotein D (gD) secreted from insect cells were analyzed using anti-HA-Tag antibodies. (C) The adhesion of gC and gD to the cell surface was analyzed by confocal microscopy. Immunostaining was performed as described in the Materials and Methods section. (D) Adhesion of gC to AK-D cells in the presence or absence of soluble heparan sulfate (HS; 1 mg/mL, 1 h at 4°C), as visualized using confocal microscopy. (E) FHV-1 entry assay in the presence or absence of soluble HS (1 mg/mL), as analyzed by qPCR. The blue color denotes nuclei, red F-actin, and green glycoproteins. Representative images are shown as maximum projections of XY stacks and XZ stacks. Scale bar: 10 μm. Data are presented as mean ± SD from three independent biological repeats with three technical replicates each. Data were analyzed with Shapiro-Wilk and Brown-Forsythe tests. One-way ANOVA with post hoc Dunnett’s test was used to determine the significance of differences between compared means. Values statistically significant are indicated by asterisks: ****P < 0.0001.
FHV-1 entry is dynamin dependent
The two primary endocytic pathways, clathrin- and caveolin-dependent, rely on dynamin II, as this GTPase plays a crucial role in vesicle formation. To study the requirement of dynamin in FHV-1 entry, we utilized two chemical inhibitors: dynasore, which inhibits dynamin GTPase activity (35, 44), and mitmab, an agent that interferes with the binding of the Pleckstrin Homology (PH) domain to phospholipids, thereby blocking dynamin recruitment (36). AK-D and FSFs cells were preincubated with non-toxic concentrations of the inhibitors (Fig. 3A) and then infected with FHV-1 in the presence of an inhibitor (entry assay). Subsequently, supernatants were collected at 8 hpi and analyzed by qPCR (Fig. 3B). Both dynasore and mitmab significantly inhibited viral replication by 82% and 72% in AK-D cells and by 65% and 75% in FSFs cells, respectively. Additionally, immunostaining of viral proteins revealed that FHV-1 replication was hampered in inhibitor-treated cells compared to the control (Fig. 3C). These results altogether indicate that FHV-1 entry is dependent on dynamin.
Fig 3
Fig 3 FHV-1 entry is dynamin-dependent. (A) First, the cytotoxicity of compounds was determined by the XTT assay. (B) For the entry assay, cells were incubated with dynasore or mitmab. Samples were collected at 8 hpi, then subjected to viral DNA isolation followed by qPCR analysis. The data were normalized to the untreated virus-infected cells. (C) Additionally, after an entry assay, infected cells were fixed and immunostained. The blue color denotes nuclei and the green is FHV-1. Representative images are shown. Scale bar: 200 μm. Data are presented as a mean ± SD from three independent experiments, each performed in triplicate or quadruplicate. Data were analyzed with Shapiro-Wilk and Brown-Forsythe tests. One-way ANOVA with post hoc Dunnett’s test was used to determine the significance of differences between compared means. Values statistically significant are indicated by asterisks: ****P < 0.0001.
FHV-1 enters the cell using pH-dependent endocytosis
To map the virus entry into the host cell, we made an effort to verify the role of endocytosis. For this, we incubated AK-D and FSFs with FHV-1 and visualized colocalization of the virus with subcellular markers at different time points p.i. (0, 30, and 60 min p.i.). First, we observed significant colocalization between FHV-1 virions and early-endosome antigen 1 (Fig. 4A), and this observation was quantified by determining Pearson’s and Mander’s coefficients. The colocalization was the most notable 30 min p.i. to decrease at 60 min p.i. slightly in AK-D and strongly in FSFs (Fig. 4B). Similar patterns were reported for other viruses that enter the cell by endocytosis (45, 46). Additionally, we incubated cells with E64d, an inhibitor of cysteine proteases, e.g., cathepsins (38), and with camostat, an inhibitor of serine proteases (37). We observed a significant decrease in viral replication by E64d, but not camostat. This indicates that FHV-1 entry and replication depend on cysteine protease activity (Fig. S2). Next, to study whether this process is pH-dependent, we incubated cells with ammonium chloride (NH4Cl), a known inhibitor of endosome acidification (31), and bafilomycin A1, which inhibits lysosomal acidification (32). The qPCR analysis revealed a profound inhibition of viral replication in AK-D and FSF cells at non-toxic concentrations (Fig. 4C and D), which suggests the requirement for endosomal acidification during the virus entry.
Fig 4
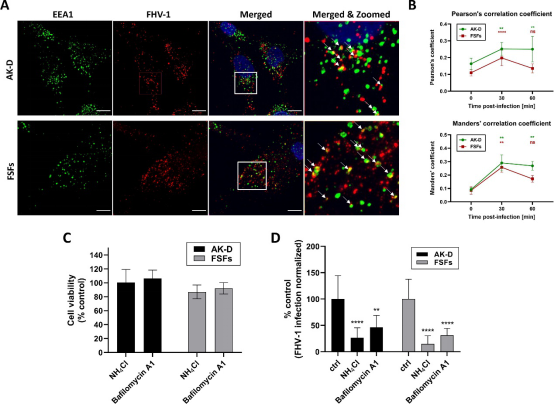
Fig 4 FHV-1 enters the cell by pH-dependent endocytosis. (A) Virus-treated cells were synchronized at 4°C for 30 min and then incubated at 37°C before being washed and fixed. Immunostaining was performed as described in the Materials and Methods section. Images present the colocalization of EEA1 and the virus after the 30-min incubation at 37°C with either FSFs or AK-D. The blue color denotes nuclei, red FHV-1, and green EEA1. Representative images are shown as maximum projections of XY stacks. Scale bar: 10 μm. Colocalization between FHV-1 and EEA1 was additionally marked by the arrows. (B) The confocal images were also quantified to estimate the colocalization determined by the Pearson’s and Mander’s coefficients for AK-D (green line) and FSFs (red line). (C) The cytotoxicity of compounds was determined by the XTT assay. (D) For the entry assay, cells were incubated with NH4Cl or bafilomycin A1. Samples were collected at 8 hpi, then subjected to viral DNA isolation followed by qPCR analysis. The data were normalized to the untreated virus-infected cells. Data are presented as a mean ± SD from three independent experiments performed in triplicate or quadruplicate. Data were analyzed with Shapiro-Wilk and Brown-Forsythe tests. One-way ANOVA with post hoc Dunnett’s test was used to determine the significance of differences between compared means. Values statistically significant are indicated by asterisks: **P < 0.01, ****P < 0.0001.
FHV-1 enters the cell via caveolin-mediated endocytosis
Next, we aimed to identify the endocytic route utilized by the FHV-1. To assess the importance of caveolae transport in FHV-1 entry, we visualized viral particles together with caveolin-1 (Fig. 5A) and performed a quantitative analysis of colocalization by determining Pearson’s and Mander’s coefficients (Fig. 5B). Robust and significant colocalization between FHV-1 and caveolin-1 was observed at 30 min p.i., increasing at 60 min p.i. in both AK-D and FSFs cells, suggesting that the virus employs caveolin-mediated endocytosis for cell entry.
Fig 5
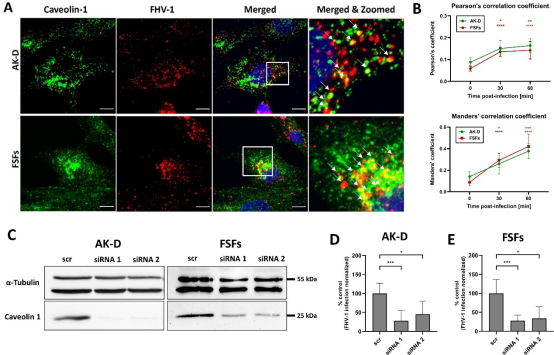
Fig 5 FHV-1 entry is caveolin-1 dependent. (A) Cells were cooled down and infected with FHV-1, the entry was synchronized at 4°C for 30 min, then cells were incubated at 37°C before being washed and fixed. Images represent the colocalization of caveolin-1 and the virus after the 30-min incubation at 37°C with either FSFs or AK-D. The blue color denotes nuclei, red FHV-1, and green caveolin-1. Representative images are shown as maximum projections of XY stacks. Scale bar: 10 μm. Colocalization between FHV-1 and caveolin was additionally marked by the arrows. (B) Quantification of the colocalization was determined by the Pearson’s and Mander’s coefficients for both AK-D (green line) and FSFs (red line), calculated for at least 10 different stacks. (C) Determination of caveolin-1 silencing efficiency using western blotting. The FHV-1 infection in AK-D (D) or FSFs (E) with silenced caveolin 1 expression was determined using qPCR. The data were normalized to the untreated virus-infected cells. Data are presented as a mean ± SD from three independent experiments, each performed in triplicate or quadruplicate. Data were analyzed with Shapiro-Wilk and Brown-Forsythe tests. One-way ANOVA with post hoc Dunnett’s test was used to determine the significance of differences between compared means. Values statistically significant are indicated by asterisks: *P < 0.05, **P < 0.01, ***P < 0.001, ****P < 0.0001.
To validate our observations, we knocked down (KD) caveolin-1 expression using siRNA silencing. Two different siRNAs were designed and used to target feline caveolin-1 and silencing efficiency was evaluated through western blot analysis (Fig. 5C). We then infected the modified cells with FHV-1 infection at an MOI of 1. Infection in caveolin-1 KD cells was significantly reduced, disregarding the model, as compared to the untreated control (Fig. 5D and E).
Finally, we employed well-known inhibitors of caveolin-mediated endocytosis: genistein, an inhibitor of caveolin-dependent endocytosis (33); nystatin, a cholesterol-sequestering agent; and filipin III, a sterol-binding agent that disrupts caveolae and caveolae-like structures (34). Cells were pre-treated with the indicated inhibitors for 1 h, then infected with FHV-1 at MOI = 1 in the presence of an inhibitor for an hour, followed by washing and overlaying with fresh medium without an inhibitor. Concurrently, compound cytotoxicity was assessed using the XTT assay (Fig. 6A). Inhibitory activity was measured using qPCR at 8 hpi (Fig. 6B). We observed inhibition of the FHV-1 replication by 78% (AK-D) and 92% (FSFs) for genistein, 75% (AK-D) and 82% (FSFs) for nystatin, and 81% (AK-D) and 94% (FSFs) for filipin. Additionally, immunostaining was performed on inhibitor-treated cells infected with FHV-1 (Fig. 6C); the same images are being used as representatives of the experimental controls as in Fig. 3C. We observed inhibition of viral entry and replication in cells treated with each inhibitor, with similar effects in AK-D and FSFs cells. These results indicate that FHV-1 entry into AK-D and FSFs cells is caveolin-1-dependent.
Fig 6

Fig 6 FHV-1 entry and replication are hampered by caveolin-1 inhibitors. (A) First, the cytotoxicity of compounds was determined by the XTT assay. (B) For the entry assay, cells were incubated with genistein, nystatin, or filipin III. Samples were collected at 8 hpi, then subjected to viral DNA isolation followed by qPCR analysis. The data were normalized to the untreated virus-infected cells. (C) Additionally, after an entry assay, infected cells were fixed and immunostained. The blue color denotes nuclei and the green is FHV-1. Representative images are shown, and the same images are being used as representatives of the experimental controls as in Fig. 3C. Scale bar: 200 μm. Data are presented as a mean ± SD from three independent experiments, each performed in triplicate or quadruplicate. Data were analyzed with Shapiro-Wilk and Brown-Forsythe tests. One-way ANOVA with post hoc Dunnett’s test was used to determine the significance of differences between compared means. Values statistically significant are indicated by asterisks: ****P < 0.0001.
FHV-1 enters FSF but not AK-D cells via clathrin-mediated endocytosis
Another commonly utilized entry pathway is clathrin-mediated endocytosis (CME) (47). To investigate the role of CME in FHV-1 entry, we visualized viral particles alongside clathrin (Fig. 7A) and conducted a quantitative colocalization analysis using Pearson’s and Mander’s coefficients (Fig. 7B). Robust and significant colocalization between FHV-1 and clathrin was observed at 15 min post-infection in FSFs cells but not in AK-D cells. This finding suggests that FHV-1 might employ different entry routes in these two models. To validate our observations, we silenced clathrin using the siRNA. Silencing efficiency was verified using a western blot (Fig. 7C). We then infected the cells with FHV-1 at an MOI of 1 and evaluated the virus replication after 8 h (single replication cycle; Fig. 7D and E). Viral replication was significantly reduced in FSFs cells but not in AK-D cells, confirming our observations.
Fig 7
Fig 7 FHV-1 entry to FSFs is clathrin-dependent but not to AK-D cells. (A) Cells were cooled down and infected with FHV-1, the entry was synchronized at 4°C for 30 min, then cells were incubated at 37°C before being washed and fixed. Images represent the colocalization of clathrin and the virus after the 30-min incubation at 37°C with either FSFs or AK-D. The blue color denotes nuclei, red FHV-1, and green clathrin. Representative images are shown as maximum projections of XY stacks. Scale bar: 10 μm. Colocalization between FHV-1 and clathrin was additionally marked by the arrows. (B) Quantification of the colocalization was determined by the Pearson’s and Mander’s coefficients for both AK-D (green line) and FSFs (red line), calculated for at least 10 different stacks. (C) Determination of clathrin silencing efficiency using western blotting. The FHV-1 infection in AK-D (D) or FSFs (E) with silenced clathrin expression was determined using qPCR. The data were normalized to the untreated virus-infected cells. Data are presented as a mean ± SD from three independent experiments, each performed in triplicate or quadruplicate. Data were analyzed with Shapiro-Wilk and Brown-Forsythe tests. One-way ANOVA with post hoc Dunnett’s test was used to determine the significance of differences between compared means. Values statistically significant are indicated by asterisks: **P < 0.01, ***P < 0.001.
DISCUSSION
It was previously described that FHV-1 attaches to HSPGs on the cell surface, which initiates the infection process (28, 29). However, little is known about the subsequent events occurring during the FHV-1 internalization. As herpesviruses utilize many different internalization routes and proteins involved in the process often are drug design targets (21), it is essential to elucidate and describe the early events during the FHV-1 internalization.
In this study, we made an effort to examine the virus entry steps. To the authors’ knowledge, it is the first study systematizing the information regarding the FHV-1 entry into the host cell. In addition, for the first time, we described the FHV-1 replication in a feline fetal lung cell line (AK-D) and primary feline skin fibroblasts (FSFs). Notably, most FHV-1 studies are performed in the CRFK cell line, a renal feline cell line. While this model allows for robust virus replication, it does not mirror the actual replication site (2, 17). Here, we developed models for the in vitro work with FHV-1, utilizing the more relevant cells. This is essential for studies on virus-host replication, as the route of entry frequently depends mainly on the cell identity; in some cases, very different entry pathways were recorded in cell lines and primary models (48). For this reason, we conducted the research using the AK-D cell line and the primary FSFs constituting the natural infection site.
First, we confirmed that HSPGs are required for successful FHV-1 attachment to the host cell, thereby serving as adhesion molecules. As expected, HS blocked the viral replication and ability to bind to the cell surface of susceptible cells, showing that HS proteoglycans are indeed responsible for virus binding on cells. This is consistent with our previous work on FHV-1 inhibitors that mimic HSPGs (41), and in line with studies on properties of the FHV-1 glycoproteins (28, 29) and other herpesviruses (18, 19, 49). Furthermore, we showed that the binding of viral glycoprotein C is inhibited by a soluble HS that confirms that gC is a protein probably responsible for viral adhesion, similar to other alphaherpesviruses (19, 50).
Next, we focused on the virus internalization routes. By different experimental approaches such as tracking single-virus particles by confocal microscopy, gene knock-down using siRNA silencing, or chemical inhibitors, we showed that FHV-1 enters AK-D cell line and FSFs by pH-dependent, dynamin-dependent, and caveolin-mediated endocytosis. Cholesterol requirement for entry was already described for herpesviruses; for example, it participates in HSV-1 entry into the human keratinocytes (26) or EHV-1 entry into brain microvascular endothelial cells (25). One may, however, remember that herpesviruses utilize a variety of different entry pathways (21). As mentioned, HSV-1 can enter keratinocytes via cholesterol-dependent endocytosis (26); to enter the human oligodendrocytic cell line, HSV employs a clathrin-dependent route (27), or to enter epithelial cells, the virus does not use either clathrin- or caveolin-mediated route (51). Therefore, by different experimental approaches, such as tracking single-virus particles by confocal microscopy and gene knock-down using siRNA silencing, we also checked whether the FHV-1 entry is clathrin-dependent. Interestingly, the virus employs CME while entering FSFs but no AK-D cells, as determined by siRNA silencing and analysis of colocalization of viral particles together with clathrin. As surprising, it is also not uncommon for viruses to use different entry pathways while entering the immortalized cell lines and primary cells. We already observed such a phenomenon in case of coronaviruses. These viruses rapidly adapt to utilize endocytic pathway to enter the immortalized cell lines, but in primary cell, viruses employ serine proteases to prime the spike protein (52).
To summarize, we mapped the FHV-1 internalization route using a feline fetal lung cell line and a model reflecting the replication site—primary feline skin fibroblasts. We confirmed the importance of HSPGs as attachment factors and showed that adhesion probably occurs through the interaction between viral gC and HSPGs. Subsequently, FHV-1 interacts with an unknown entry receptor and is internalized by dynamin-dependent, pH-dependent, and caveolin-1-mediated endocytosis. In the case of FSFs, it can also occur via a clathrin-dependent route. This study provides key insights into the first step of FHV-1 infection, viral entry into cells, and new infection models.
ACKNOWLEDGMENTS
AS's work was supported by the Foundation for Polish Science (FNP). Graphical abstract was created with BioRender.com.
This work was supported by the Ministry of Science and Higher Education, Poland within the Diamond Grant funding scheme (0200/DIA/2018/47) to AS, and DURABLE (Delivering a Unified Research Alliance of Biomedical and public health Laboratories against Epidemics) project funded by the European Commission within the EU4Hlth initiative under grant no.101102733.
The authors declare no conflict of interest.
The funders had no role in the preparation of the manuscript.